Research Vision: The advent of molecular biology and particularly of genomic sciences has ensured that there is no shortage of medicinally relevant biological systems and proteins to study. This represents an area of organic chemistry of growing importance, which is increasingly guided by biology in bridging the divide between chemistry and biology and in determining the most suitable points of attack for future medicines and materials. Using concepts and methods taken from both chemistry and biology and through the powerful self-assembly of predictable supramolecular entities, we are aiming to lock the multi-component ensembles into chemically or biologically interesting conformations and subsequently apply them to address varying challenging issues and to find important applications in nanotechnology at the interface of chemistry, materials and biology. It is our sincere hope that the efforts and dedication put in these therapeutically or environmentally significant research endeavors eventually would help shape the course of DNA sequencing, drug discovery, water purification by some measures to better human welfare and health.
Specifically, building upon our past and current research accomplishments and findings on developing artificially created transmembrane channels and with additional inspirations from Nature, we will prioritize and build what we consider as the most important to address key challenges and practical uses in the field of artificial channels. Our further strategic research roadmap entails the following Research Projects, which are being actively pursued together with many others in our pragmatic interdisciplinary research efforts on artificial membrane transporters and other chemistry frontiers.
Unconventional transporters that do not utilize channel or carrier mechanisms for ion transport
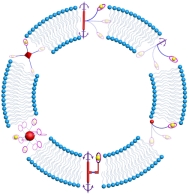
We recently demonstrated that macroscopic swing and ion-fishing mechanisms can be applied in the microscopic world to facilitate ion transport across lipid membrane (For a recent review by us, see: Acc. Chem. Res. 2022, 55, 1148; For molecular swing, see: Angew. Chem. Int. Ed. 2019, 58, 8034; For molecular ion fishers, see: J. Am. Chem. Soc. 2019, 141, 9788; For molecular ball, see: J. Am. Chem. Soc. 2020, 142, 21082; For molecular tetrahedron, see: CCS Chem. 2020, 2, 2269For ion swimmer, see: Nano Lett. 2021, 21, 1384; For molecular rotor, see: Sci. China Chem. 2021, 64, 2154). Shall we just stop here? Certainly we shall not for two-fold reasons. On the one hand, each new type of transporter by its own right is highly modular in structure, enabling an evolutionary approach with a parallel synthesis of library members to be applied for rapid discovery of ion channels with new properties. On the other hand, in addition to swings, fishers, rotors, etc, macroscopic objects also consist of many others, including propeller, pump, mover and windmill. Can these macroscopic functions be mimicked at the molecular level for ion transport?
Synthetic water channels for water purification
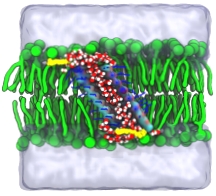
The fact that 4 billion people globally suffering from severe water scarcity has made the reverse-osmosis (RO) membrane technique the dominant process for clean water production, currently generating 100 million tons of desalinated water per day from the Oceans. Its high energy consumption however limits its widespread adoption, but in the meantime also motivated researchers to develop synthetic water channels for blending into RO membrane to decrease the water desalination pressure and consequently reduce the energy consumption. For this purpose, unifying high water permeability and selectivity in one simplified water channel system is an indispensable prerequisite, which is and so far proves to be challenging. Recently, we made some encouraging discovery, via a “sticky” end-mediated construction strategy (see: J. Am. Chem. Soc. 2014, 136, 14270 and Acc. Chem. Res. 2016, 49, 922), of a novel synthetic water channel that displays high water transport (~ 3 x 10⁹ water molecules/s) and high rejection of salts such as NaCl and KCl. (J. Am. Chem. Soc. 2020, 142, 10050; Angew. Chem. Int. Ed. 2020, 59, 13328), and has met application parameter requirements. More recently, we devised another completely different and novel type of polymer-based water channels that increases the water transport permeability by 8-fold to 2.7 x 10¹º water molecules/s (Nat. Nanotech. 2021, 16, 911). While striving to further improve their water transport properties, we are now attempting to find different ways of blending these powerful synthetic water channels into RO membrane or making of novel types of water channel-containing non-RO membrane for water purification purpose, which could form the basis for next-generation water purification technology with less energy consumption and better performance.
Highly selective synthetic ion/proton channels
A hallmark of protein channels is their high selectivity in transporting related species across cellular membranes via evolutionarily selected transmembrane pathways. Some notable examples include aquaporins that are super permeable to water molecules (10⁹ molecules per second) but strictly prevent the passage of ions and even protons, the K+- channel KcsA that displays a K+/Na+ selectivity of 104, and the M2 proton channel that is at least 105-fold more selective for protons over other monovalent cations. While these extraordinarily high transport selectivities are crucial for proper cellular functions, including maintaining an ion concentration gradient and regulating membrane potential, they are extremely difficult to replicate in artificially developed species-transporting channels. Over the past few years, we have developed some synthetic versions, enabling highly selective transport of K+ (J. Am. Chem. Soc. 2017, 139, 12338; Angew. Chem. Int. Ed. 2020, 59, 1440), Cl- (J. Am. Chem. Soc. 2018, 140, 8817) and I- (Angew. Chem. Int. Ed. 2020,59, 4806) ions as well as protons (Angew. Chem. Int. Ed. 2022, 61, e202200259). These are the areas that continue to capture our attention.
Combinatorial chemistry for rapid optimization of channel’s ion transport selectivity
Combinatorial chemistry was introduced into the field of artificial channels first by us in 2017 to evolve synthetic potassium channels with high K+/Na+ selectivity of 9.8 (J. Am. Chem. Soc. 2017, 139, 12338). There is still much to do with this combinatorial chemistry. Some of its already demonstrated yet unpublished applications include increase of K+/Na+ selectivity to 20.1 and development of amino acid-specific transporters. Along the same line, we would be interested to further increase K+/Na+ selectivity or investigate if Na+/K+ selectivity can reach an unprecedented value of 10.
Mechanosensitive ion channels
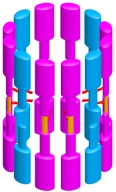
Mechanosensitive channels are present in the membranes of bacteria, archaea, and eukarya, sensing touch, hearing and balance, as well as participating in cardiovascular regulation and osmotic homeostasis (e.g. thirst). One such type of mechano-channels found in bacteria is cholesterol-dependent channels, with ion transporting activity increasing with increasing amounts of cholesterol so as to attack the eukaryotic cells that contain cholesterol in the membrane, but not bacterial cells that do not contain cholesterol. Inspired by this, we recently designed and experimentally demonstrated an unprecedented class of mechanosensitive ion channels that perfectly mimic those found in bacterial (See: Nat. Commun. 2022, 13, 5985). This field, which thus far has remained limitedly studied, is worth further and systematic investigations in the future.
Gated ion channels
Naturally occurring protein channels can be gated on and off by voltage, ligands, light, enzymes, etc. These are the forefront areas we have not touched yet due to a lack of manpower but will be pursued when possible.
Active transporters
Active transport refers to moving ions or molecules across a cell membrane into a region of higher concentration, an energy-consuming process that has to be assisted by enzymes. In Nature, sources of energy for primary active transport include metabolic energy as used by ATPases for Na+/K+ exchange,redox energy as used by the mitochondrial electron transport chain for proton movement, and light energy as used by photosynthesis proteins to create a proton gradient. In light of these inspirations, we will look into means of tackling this challenging research forefront via creating synthetic versions of naturally occurring active transports.
Synthetic precision nanochannels for DNA sequencing
In 2004, the US National Institutes of Health proposed a $1,000 per human genome challenge. “How much will new technologies lower the cost of sequencing?” was one of the 125 big questions for the next quarter century proposed in Science 2005, 309, 78. Among emerging sequencing technologies, nanopore sequencing, which combines the potential for ultra-long read length (104 – 106 bases) at high speed without costly procedures (e.g., biochemical labelling, PCR amplification) while extracting the maximum amount of information from the minimum amount of materials, might offer a solution to this daunting challenge.Recently, Oxford Nanopore Technologies (ONT) released a portable nanopore sequencer MinION, which is based on protein nanopores such as α-hemolysin and phi29 DNA polymerase (DNAP). Its high error profile certainly suggests a need for alternative nanopore sensing devices likely made of synthetic nanopores with precision to read DNA sequences at single-nucleotide resolution.In this project, we will be developing an entirely new class of synthetic nanopores with modularly tunable macrocyclic or helically folded interior cavities as an alternative to the existing natural or solid state nanopores. These macrocyclic or helical nanopores feature readily modifiable interior surface to potentially enable binding and transport of four DNA nucleotides (A, T, G and C) at precisely controlled speeds, and eventually may allow reading of DNAs at single-nucleotide resolution. Once successful, this new technology would be a revolutionary alternative to the current nanopore system, enabling rapid and reliable DNA sequencing with ultra-long reads while making whole-genome studies available to the general population at a low cost. Besides DNA sequencing, this technology can also be used for RNA sequencing and identification of epigenetic modification, such as base methylation. This will have far-reaching benefits for public health, particularly in early disease diagnostics, understanding of genetic causes of diseases, personalized medicine and rapid identification of pathogens.